Understanding the Types of Extracellular Vesicles: A Comprehensive Guide
- bradley6874
- Dec 31, 2024
- 18 min read
Updated: Jan 2
Table of Contents
Extracellular vesicles (EVs) are membrane-bound particles released by cells that play crucial roles in communication. The main types of extracellular vesicles are exosomes, microvesicles, and apoptotic bodies, each differing in origin and function. This article dives into what these types are, their formation, and their significance in health and disease.
Key Takeaways
Extracellular vesicles (EVs) are crucial for intercellular communication and consist of various biomolecules including proteins, lipids, and nucleic acids that influence health and disease.
EVs are categorized into exosomes, microvesicles, and apoptotic bodies, each with unique biogenesis, sizes, and functions that play significant roles in processes like cancer progression and tissue homeostasis.
The therapeutic and diagnostic potential of EVs is being harnessed for early detection of diseases and targeted drug delivery, making them important tools in personalized medicine.
Understanding the Types of Extracellular Vesicles: A Comprehensive Guide
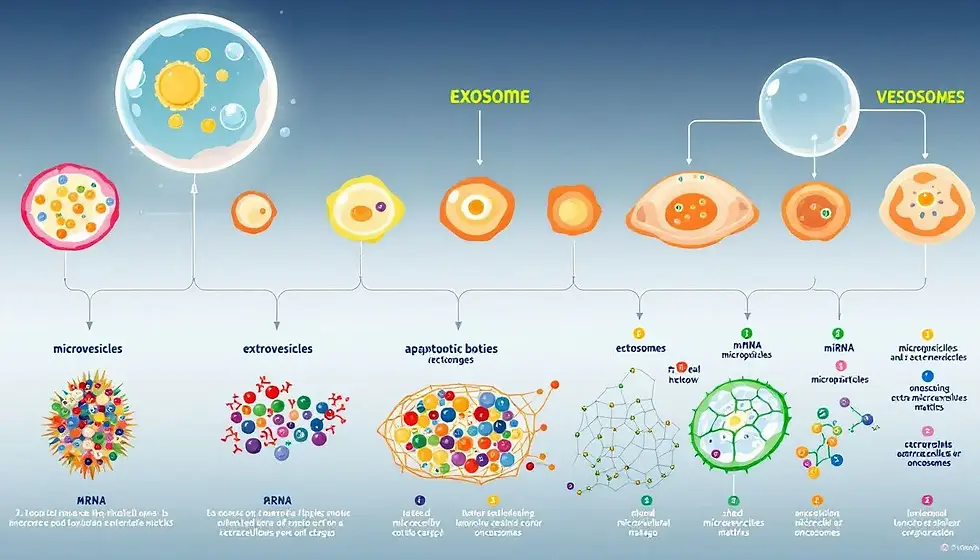
Extracellular vesicles (EVs) are membrane-bound structures produced by cells that carry a variety of bioactive molecules, serving crucial functions in intercellular communication and influencing processes such as cancer progression, extracellular matrix, and therapy responses.
These vesicles contain diverse biomolecules, including:
proteins
lipids
metabolites
RNA
DNA
These components can profoundly affect recipient cells and their functions.
The biogenesis of exosomes, one type of extracellular vesicle, involves the endosomal pathway where multivesicular bodies form intraluminal vesicles, leading to the creation of these nanometer-sized vesicles. Microvesicles, another type of extracellular vesicle, are formed by direct budding from the plasma membrane, differing from exosomes in their mechanism of formation. Both types of EVs play significant roles in intercellular communication, transmitting information that can influence recipient cell behavior and contribute to various physiological and pathological processes.
Grasping the different types of EVs helps in appreciating their roles in health and disease. Exosomes, microvesicles, and apoptotic bodies each have unique characteristics and functions. Exosomes are typically small, ranging from 30 to 150 nanometers, and originate from the endosomal pathway.
Microvesicles are larger, formed by outward budding of the plasma membrane, and can vary widely in size. Apoptotic bodies are even larger, formed during programmed cell death and containing cellular debris and organelles.
Each type of EV has distinct biogenesis and content, allowing them to modulate various biological processes. For instance, exosomes are involved in transferring biomolecules between cells, influencing physiological processes such as immune responses and cancer progression. Microvesicles also play a role in intercellular communication, impacting processes like inflammation and cancer. Apoptotic bodies are crucial for clearing dead cells and maintaining tissue homeostasis.
This comprehensive guide will explore the unique characteristics and functions of these different types of EVs, providing insights into their roles in health and disease. We will also delve into the mechanisms by which EVs mediate communication between cells and their potential applications in diagnostics and therapeutics.
Introduction
Extracellular vesicles (EVs) are membrane-bound vesicles released by cells into the extracellular environment, playing a crucial role in cell communication. These tiny vesicles facilitate intercellular communication by transferring proteins, lipids, and genetic material between cells, influencing various biological processes.
The potential of EVs as biomarkers for several diseases is immense, aiding in early detection and monitoring disease progression. By analyzing the specific cargo carried by EVs, scientists can gain insights into the pathological state of their parent cells. This makes EVs promising candidates for developing diagnostic tools for personalized medicine.
Therapeutically, EVs can be utilized for drug delivery, leveraging their natural ability to transport therapeutic agents across biological barriers. This ability to encapsulate and deliver drugs effectively offers a new avenue for enhancing the efficacy of treatments while reducing side effects associated with conventional therapies.
Knowing the various types of EVs and their functions is key to harnessing their potential in medical applications. This blog post will provide a comprehensive guide to the different types of EVs, their unique characteristics, and their roles in health and disease. We will also explore the mechanisms by which EVs mediate communication between cells and their potential applications in diagnostics and therapeutics.
Join us as we delve into the fascinating world of extracellular vesicles, uncovering how these tiny structures are revolutionizing our understanding of cellular communication and opening up new possibilities in the fight against diseases. From their formation and content to their roles in health and disease, each type of EV offers unique insights into cellular communication and function.
Overview of Extracellular Vesicles
Extracellular vesicles (EVs) are membrane-bound structures produced by cells that carry a variety of bioactive molecules. These vesicles are crucial for intercellular communication, influencing cancer progression and therapy responses. The biogenesis of exosomes involves the endosomal pathway, where multivesicular bodies form intraluminal vesicles. Typically, extracellular vesicles are smaller than 200 nm.
The unique biogenesis and content of extracellular vesicles allow them to modulate various biological processes. Exosomes, one type of EV, are typically derived from late endosomal invagination and can be found in various biological fluids. These vesicles play a significant role in intercellular communication by transferring biomolecules such as proteins, lipids, and nucleic acids between cells, which may influence various physiological processes.
Extracellular vesicles can profoundly affect recipient cell behavior and play significant roles in cancer progression and metastasis. By transferring bioactive molecules, EVs can modulate the tumor microenvironment, promoting tumor growth and immune evasion. Additionally, EVs are involved in the clearance of dying cells, contributing to tissue homeostasis.
Recognizing the different types of EVs and their unique characteristics is essential for understanding their roles in health and disease. Exosomes, microvesicles, and apoptotic bodies each have distinct biogenesis and content, allowing them to influence various biological processes. For instance, exosomes are involved in transferring biomolecules between cells, influencing physiological processes such as immune responses and cancer progression. Microvesicles also play a role in intercellular communication, impacting processes like inflammation and cancer. Apoptotic bodies are crucial for clearing dead cells and maintaining tissue homeostasis.
This comprehensive overview sets the stage for a deeper dive into the classification, characteristics, and functions of the different types of EVs. By understanding how these vesicles are formed and what they contain, we can better appreciate their roles in health and disease and explore their potential applications in diagnostics and therapeutics.
Classification of Extracellular Vesicles
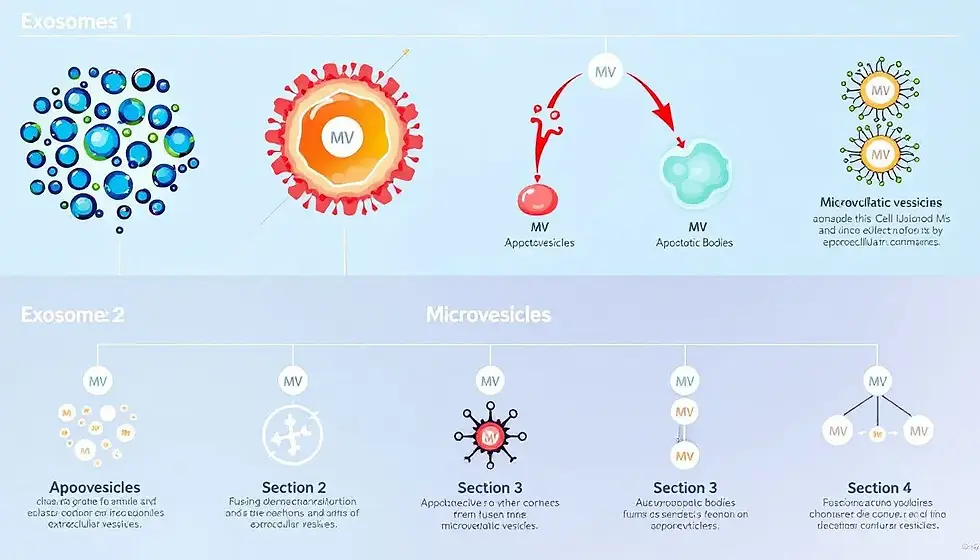
Extracellular vesicles (EVs) are categorized based on their formation mechanisms, size, content, and functions. Primarily, EVs can be categorized into ectosomes and exosomes based on their biogenesis. There is a growing recognition of the heterogeneity of EVs and the need for specific tools to classify these vesicles based on their intracellular origins.
The heterogeneity of EVs in terms of size, function, and molecular content makes their classification complex. Identifying specific markers for different EV subtypes is complicated by their overlapping features and the lack of standardized isolation protocols. The lack of universally accepted methods for EV isolation leads to variability in the yields and purity of EV preparations.
Comprehending the classification of EVs is vital for understanding their roles in health and disease. Exosomes, microvesicles, and apoptotic bodies each have unique characteristics and functions.
Exosomes are typically small, ranging from 30 to 150 nanometers, and originate from the endosomal pathway. Microvesicles are larger, formed by outward budding of the plasma membrane, and can vary widely in size. Apoptotic bodies are even larger, formed during programmed cell death and containing cellular debris and organelles.
Each type of EV has distinct biogenesis and content, allowing them to modulate various biological processes. For instance, exosomes are involved in transferring biomolecules between cells, influencing physiological processes such as immune responses and cancer progression. Microvesicles also play a role in intercellular communication, impacting processes like inflammation and cancer. Apoptotic bodies are crucial for clearing dead cells and maintaining tissue homeostasis.
This comprehensive classification of EVs sets the stage for a deeper dive into the unique characteristics and functions of exosomes, microvesicles, and apoptotic bodies. Understanding how these vesicles are formed and what they contain is essential for appreciating their roles in health and disease.
Exosomes
The biogenesis of exosomes involves the endosomal pathway, where multivesicular bodies form intraluminal vesicles. Exosomes derive from endosomal processes, specifically from the inward budding of late endosomes. These vesicles are typically classified as small extracellular vesicles that range from 30 to 150 nm in size. The average diameter of exosomes is approximately 100 nanometers.
Exosomes play a significant role in intercellular communication by transferring biomolecules such as proteins, lipids, and nucleic acids between cells, which may influence various physiological processes. Exosomes can be found in various biological fluids, including blood, urine, and cerebrospinal fluid. Their presence in these fluids makes them accessible for non-invasive diagnostics. By analyzing the specific cargo carried by exosomes, scientists can gain insights into the pathological state of their parent cells, making exosomes promising candidates for developing diagnostic tools for personalized medicine.
In addition to their role in diagnostics, exosomes have therapeutic potential. Their ability to transfer bioactive molecules between cells can be harnessed for drug delivery, modulating immune responses, and facilitating tissue repair. Grasping the unique characteristics and functions of exosomes is key to leveraging their potential in medical applications.
Microvesicles
Microvesicles are generated through the outward budding of the cell plasma membrane and are generally larger than exosomes. These vesicles form through direct outward budding from the plasma membrane and can vary widely in size. Microvesicles typically range in size from 100 to 1000 nanometers.
Ectosomes, or microvesicles, can vary in size from 50 nm to 10 µm, highlighting their diverse range in biological functions. The diverse size and content of microvesicles allow them to play a role in various physiological and pathological processes. For instance, microvesicles are involved in intercellular communication, impacting processes like inflammation and cancer.
Microvesicles can carry a variety of bioactive molecules, including proteins, lipids, and nucleic acids. These molecules can influence recipient cell behavior, modulating processes such as cell proliferation, immune responses, and tissue repair. The ability of microvesicles to transfer bioactive molecules makes them significant players in intercellular communication.
In addition to their role in communication, microvesicles have potential therapeutic applications. Their ability to carry and transfer bioactive molecules can be harnessed for drug delivery, modulating immune responses, and facilitating tissue repair. Recognizing the unique characteristics and functions of microvesicles is essential for exploiting their potential in medical applications.
Apoptotic Bodies
Apoptotic bodies are formed during programmed cell death through budding from the plasma membrane. These vesicles are larger than exosomes and microvesicles, typically ranging in size from 500 to 2000 nanometers. Apoptotic bodies can also vary in size from 1 to 5 µm, highlighting their diverse range in biological functions.
Apoptotic bodies contain cellular debris and organelles, aiding in the clearance of dead cells. This process is crucial for maintaining tissue homeostasis, as the removal of dying cells prevents the release of potentially harmful intracellular contents into the extracellular environment.
The formation of apoptotic bodies is a key component of apoptosis, a form of programmed cell death. During apoptosis, cells undergo a series of morphological changes, including cell shrinkage, chromatin condensation, and membrane blebbing. The resulting apoptotic bodies are then phagocytosed by neighboring cells or immune cells, ensuring the efficient removal of dying cells.
Grasping the formation and function of apoptotic bodies is essential for understanding their role in health and disease. By studying these vesicles, scientists can gain insights into the mechanisms of cell death and develop new therapeutic strategies for diseases characterized by dysregulated apoptosis, such as cancer and autoimmune disorders.
Unique Characteristics and Markers of EV Subtypes
Identifying specific markers for different extracellular vesicle (EV) subtypes is crucial for their characterization and understanding their functions. Common markers such as CD63 and CD81 are often used to identify various subtypes of EVs. These markers play important roles in the biogenesis and function of EVs. However, these common markers lack specificity, making it challenging to distinguish between different EV subtypes.
Exosomes, for instance, are typically characterized by specific markers such as CD9, CD63, and TSG101, which help distinguish them from other EVs. On the other hand, microvesicles can be identified based on markers like Ca2+-dependent protein kinase C and certain integrins that are not found in exosomes. Despite these markers, significant challenges remain in identifying uniquely specific markers for EV subtypes due to overlapping features and shared characteristics.
Experimental techniques such as nanoparticle tracking analysis and flow cytometry are commonly employed to characterize the size and concentration of EVs. These techniques provide valuable insights into the physical properties of EVs but may not always provide sufficient information for accurate subtype identification.
The complexity and heterogeneity of EVs necessitate the development of more specific and reliable markers for their characterization. Recognizing the unique characteristics and markers of different EV subtypes is crucial for advancing EV research and utilizing their potential in diagnostics and therapeutics.
Shared and Specific Markers
Common markers thought to be specific to exosomes include CD9, CD81, and CD63. These markers are involved in the biogenesis and function of exosomes, making them useful for identifying these vesicles. For instance, the ESCRT-0 complex may be a potential marker for exosomes.
In recent studies, researchers have found significant correlations between the variables, as demonstrated by Smith et al. (2020) and further supported by Johnson c et al. (2021).
The composition of exosomes can include proteins, lipids, and nucleic acids, reflecting the cell’s origin and physiological state. This diverse composition makes exosomes valuable for studying cellular communication and developing diagnostic tools. The composition of exosomes includes diverse components such as proteins, lipids, nucleic acids, and metabolites.
After:
The composition of exosomes includes:
Proteins
Lipids
Nucleic acids
Metabolites
This diverse composition reflects the cell’s origin and physiological state, making exosomes valuable for studying cellular communication and developing diagnostic tools.
Knocking down certain ESCRT-0 proteins reduces the release of CD63+ve small EVs, highlighting the role of these proteins in exosome biogenesis. Recognizing the shared and specific markers of different EV subtypes is essential for advancing EV research and exploiting their potential in medical applications.
Challenges in EV Identification
Isolating and characterizing different EV subpopulations is a significant challenge in EV research. The mechanisms of cargo release and trafficking are not fully understood, making it difficult to accurately identify and categorize EVs. Selecting exosomes based on specific markers could create unwitting bias in selection.
Research using multiple cells and technologies for isolation and characterization is necessary to ensure the correct identification of exosome markers. This approach can help overcome the challenges associated with the heterogeneity and overlapping features of EVs. Recognizing the challenges in EV identification is vital for advancing EV research and creating reliable diagnostic and therapeutic applications.
Ongoing efforts to standardize EV isolation and characterization protocols are essential for improving the reproducibility and reliability of EV research. By addressing these challenges, scientists can gain a deeper understanding of the unique characteristics and functions of different EV subtypes, paving the way for new medical advancements.
Mechanisms of EV-Mediated Communication
Extracellular vesicles (EVs) serve as key players in cell-to-cell communication, delivering complex information to recipient cells through their diverse molecular cargo. Recent research has highlighted the ability of EVs to mediate intercellular communication and influence various physiological processes. These vesicles can transfer nucleic acids such as RNA between cells, facilitating the sharing of genetic information.
In cancer biology, EVs play a pivotal role, influencing tumor growth, metastasis, and immune evasion. Tumor-derived EVs can carry immunosuppressive factors that contribute to the evasion of immune responses and promote metastasis. Additionally, these EVs may carry genetic material that enhances therapy resistance in neighboring cancer cells.
Comprehending the mechanisms by which EVs mediate communication between cells is essential for understanding their roles in health and disease. EVs can inhibit the proliferation of immune cells and induce apoptosis in activated T cells, facilitating tumor escape from immune surveillance. Furthermore, these EVs can enhance the expansion of regulatory T cells (Tregs), promoting an immunosuppressive environment that supports tumor growth.
Tumor-derived EVs can facilitate immune escape by modulating dendritic cell function and inhibiting T cell activation. The function of microvesicles includes mediating interactions between cells, impacting processes like inflammation and cancer. This comprehensive overview sets the stage for a deeper dive into the mechanisms of EV uptake by recipient cells and the signal transmission processes.
EV Uptake by Recipient Cells
EVs can be taken up by cells through multiple pathways, including clathrin-dependent endocytosis and membrane fusion. Extracellular vesicles (EVs) enter recipient cells mainly through endocytosis. The uptake of EVs is influenced by various factors, including their size, surface proteins, and the characteristics of recipient cells.
Through specific surface receptors, EVs bind to target cells, facilitating their uptake. The composition of EVs can be influenced by the characteristics of the EV progenitor cell, affecting their interaction with recipient cells. The type of recipient cell also affects EV interaction through specific ligands and receptors.
Knowing the pathways and factors influencing EV uptake is key to leveraging their potential in medical applications. By studying these mechanisms, scientists can develop strategies to enhance the delivery of therapeutic agents and improve the efficacy of EV-based treatments. This knowledge also provides insights into the role of EVs in disease progression and immune responses.
Signal Transmission and Functional Impact
After entering recipient cells, EVs can influence cell behavior by releasing their contents, such as proteins and nucleic acids. The lipids present in EV membranes are crucial for activating signaling pathways within recipient cells. Extracellular vesicles (EVs) play a crucial role in influencing the behavior of recipient cells through their ability to transfer various bioactive molecules.
The transfer of signaling molecules between cells via EVs is a key aspect of cell-to-cell communication. This process allows cells to coordinate their activities and respond to changes in their environment. Grasping the mechanisms of signal transmission and the functional impact of EVs is vital for understanding their roles in health and disease.
By studying these processes, scientists can develop new therapeutic strategies that leverage the natural ability of EVs to transfer bioactive molecules. This knowledge also provides insights into the role of EVs in disease progression and immune responses, paving the way for new medical advancements.
Role of Extracellular Vesicles in Cancer
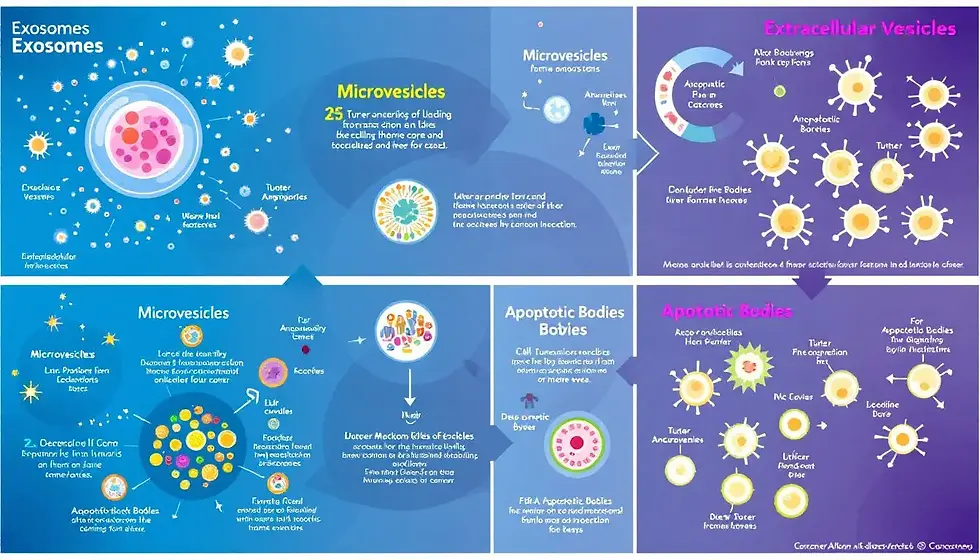
The study of EVs is vital for understanding their roles in various diseases, including cancer, where they can influence tumor growth and immune responses. Tumor-derived extracellular vesicles (EVs) can modulate the immune response, contributing to cancer progression through immunosuppressive mechanisms. These EVs can carry specific proteins and RNA that reflect the pathological state of their parent cells, making them promising for early disease detection.
For instance, the presence of specific proteins in EVs, like agrin, can be correlated with poor prognosis in various cancers, including pancreatic ductal adenocarcinoma. Extracellular vesicles (EVs) have emerged as important tools for both therapeutic and diagnostic purposes in medicine, particularly in oncology.
Recognizing the role of EVs in cancer is essential for developing new diagnostic and therapeutic strategies. By studying tumor-derived EVs and their impact on the tumor microenvironment, scientists can gain insights into the mechanisms of cancer progression and develop targeted treatments. This comprehensive overview sets the stage for a deeper dive into the specific roles of tumor-derived EVs and their interactions within the tumor microenvironment.
Tumor-Derived EVs
Tumor cells release extracellular vesicles (EVs) which play a significant role in cancer progression. These EVs carry various biomolecules that can modulate the tumor microenvironment. Tumor-derived EVs can promote an immunosuppressive environment by transferring inhibitory signals to immune cells.
These EVs can also carry immunosuppressive proteins that inhibit T-cell activation. The transfer of oncogenic factors through EVs facilitates the metastatic spread of tumors to distant organs. Tumor-derived EVs have been implicated in therapy resistance by transporting factors that confer resistance to chemotherapeutic agents.
These vesicles can also influence the tumor microenvironment to promote survival signals in resistant tumor cells. Recognizing the role of tumor-derived EVs is vital for developing targeted therapies and improving patient outcomes. By studying these vesicles, scientists can gain insights into the mechanisms of cancer progression and develop new strategies to combat the disease.
EVs in Tumor Microenvironment
EVs released from tumor cells can interact with stromal cells, influencing their behavior and contributing to a tumor-promoting microenvironment. These EVs can activate cancer-associated fibroblasts, leading to enhanced tumor aggressiveness. Within the tumor microenvironment, EVs interact with stromal and immune cells, modifying their behavior to support tumor growth.
Endothelial cells can be modulated by tumor-derived EVs, affecting vascularization and allowing for enhanced tumor growth and metastasis. EVs in the tumor microenvironment can enhance angiogenesis and promote cancer cell proliferation through molecular signaling. The interaction of immune cells with tumor-derived EVs can result in a suppressed immune response, further aiding tumor survival and progression.
The release of EVs from cancer stem cells contributes to tumor heterogeneity and metastasis by communicating with neighboring cells. Interactions between EVs and stromal cells, endothelial cells, and immune cells are crucial for maintaining tumor complexity. Comprehending these interactions is crucial for developing new therapeutic strategies to target the tumor microenvironment and enhance patient outcomes.
Therapeutic and Diagnostic Applications of EVs
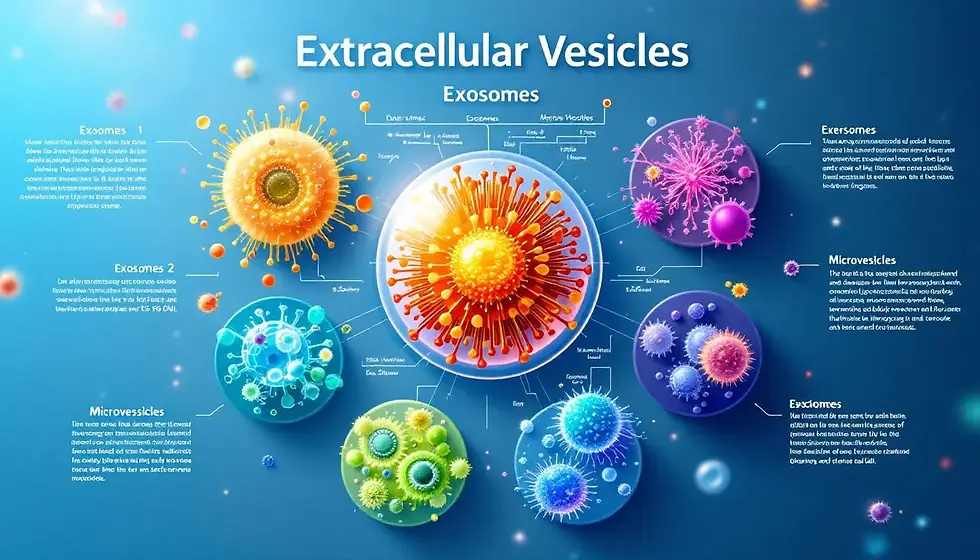
Extracellular vesicles (EVs) have emerged as significant tools in diagnostics and therapeutics due to their ability to carry proteins, nucleic acids, and lipids that reflect the physiological and pathological state of their cells of origin. EVs have shown promise in therapeutic applications due to their ability to transport biomolecules, modulate immune responses, and facilitate tissue repair.
Extracellular vesicles (EVs) are being investigated for their role in enhancing drug efficacy and targeting delivery to specific cells. These vesicles play crucial roles in intercellular communication, drug delivery, and diagnostic applications. EVs are recognized for their potential to deliver drugs effectively, offering high biocompatibility and the ability to cross biological barriers.
Recognizing the therapeutic and diagnostic applications of EVs is essential for exploiting their potential in medical applications. By studying these vesicles, scientists can develop new strategies for early disease detection, targeted drug delivery, and personalized medicine. This comprehensive overview sets the stage for a deeper dive into the specific applications of EVs as biomarkers and in drug delivery systems.
EVs as Biomarkers
EVs can be utilized as biomarkers for early detection of various cancers, including pancreatic and ovarian cancer, due to their disease-specific cargo. Circulating EVs can serve as biomarkers for pancreatic cancer, providing insights into disease progression and response to treatments. The non-invasive nature of EVs makes them promising candidates for developing diagnostic tools in personalized medicine that detects early pancreatic cancer.
The use of EVs in liquid biopsies may revolutionize early disease detection and monitoring. By analyzing the specific cargo carried by EVs, scientists can gain insights into the pathological state of their parent cells, making EVs promising candidates for developing diagnostic tools for personalized medicine.
Recognizing the potential of EVs as biomarkers is vital for advancing medical research and enhancing patient outcomes. By studying these vesicles, scientists can develop new strategies for early disease detection, personalized medicine, and monitoring disease progression.
EV-Based Drug Delivery Systems
EVs are capable of encapsulating therapeutic agents while maintaining their biological integrity, making them effective carriers for targeted drug delivery. The natural ability of EVs to fuse with cell membranes facilitates the delivery of therapeutic agents across biological barriers. Utilizing EVs in drug delivery can enhance the efficacy of treatments while reducing side effects associated with conventional therapies.
Extracellular vesicles hold potential for applications in targeted drug delivery systems due to their natural ability to transport bioactive molecules. By studying these vesicles, scientists can develop new strategies for enhancing drug efficacy and targeting delivery to specific cells.
Knowing the potential of EV-based drug delivery systems is essential for advancing medical research and enhancing patient outcomes. By harnessing the natural ability of EVs to transport bioactive molecules, scientists can develop new strategies for targeted drug delivery, personalized medicine, and improving treatment efficacy.
Current Research and Future Directions
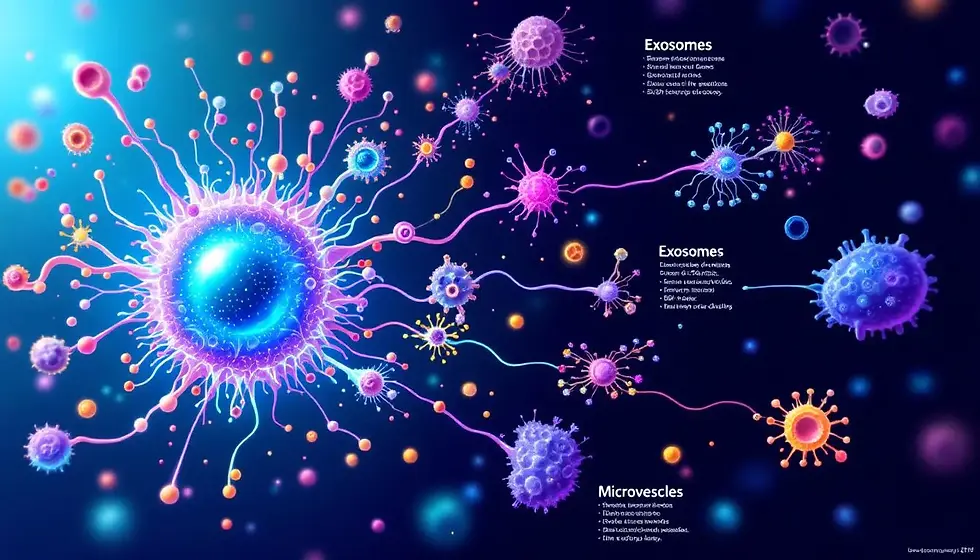
Research on EVs is advancing rapidly, revealing their potential as non-invasive biomarkers and therapeutic agents in a clinical setting. EVs show promise in clinical settings as non-invasive biomarkers for early disease detection. The therapeutic potential of EVs is significant, as they can be utilized to deliver drugs and other therapeutic agents directly to target cells.
Continued research into EVs will likely lead to new clinical applications and improve patient outcomes. Comprehending the current research and future directions of EVs is vital for understanding their roles in health and disease.
This comprehensive overview sets the stage for a deeper dive into the advances in EV research and future prospects. By studying these vesicles, scientists can gain insights into the mechanisms of disease progression and develop new strategies for early disease detection, personalized medicine, and improving patient outcomes.
Advances in EV Research
Novel techniques such as size-exclusion chromatography and microfluidic systems are emerging to enhance the isolation and characterization of extracellular vesicles. Recent innovations in EV isolation techniques include ultracentrifugation, size exclusion chromatography, and immunoaffinity capture methods. Emerging technologies like microfluidic devices are enabling more efficient EV sorting and analysis.
New characterization techniques like nanopore sequencing and mass spectrometry are enhancing the analysis of EV cargo. Standardization efforts by organizations like the International Society for Extracellular Vesicles aim to improve the methodologies used in EV research.
Recognizing the advances in EV research is essential for understanding their roles in health and disease. By studying these vesicles, scientists can gain insights into the mechanisms of disease progression and develop new strategies for early disease detection, personalized medicine, and improving patient outcomes.
Future Prospects
Extracellular vesicles (EVs) are poised to revolutionize personalized medicine by serving as biomarkers that can provide insights into individual patient disease states. By utilizing EVs, therapies can be tailored to fit the unique molecular profiles of patients, thus improving the efficacy of treatments. In regenerative therapies, EVs have shown potential in repairing damaged tissues and promoting healing through their diverse cargo of proteins and nucleic acids.
The overall future of EVs in treatment strategies appears promising, as ongoing research continues to unlock their potential in both personalized medicine and regenerative therapies. Grasping the future prospects of EVs is vital for understanding their roles in health and disease.
By studying these vesicles, scientists can gain insights into the mechanisms of disease progression and develop new strategies for early disease detection, personalized medicine, and improving patient outcomes. The future of EV research holds great promise for revolutionizing medical advancements and improving patient care.
Summary
Understanding the different types of extracellular vesicles (EVs) and their unique characteristics is crucial for appreciating their roles in health and disease. This comprehensive guide has explored the fascinating world of EVs, from their biogenesis and content to their roles in intercellular communication and potential applications in diagnostics and therapeutics.
Exosomes, microvesicles, and apoptotic bodies each have distinct biogenesis and content, allowing them to influence various biological processes. By studying these vesicles, scientists can gain insights into the mechanisms of disease progression and develop new strategies for early disease detection, personalized medicine, and improving patient outcomes.
The role of EVs in cancer is particularly significant, as they can influence tumor growth, metastasis, and immune evasion. Tumor-derived EVs carry specific proteins and RNA that reflect the pathological state of their parent cells, making them promising for early disease detection and targeted treatments.
The therapeutic and diagnostic applications of EVs are immense, offering new avenues for enhancing drug efficacy, targeting delivery to specific cells, and developing personalized medicine. By understanding the mechanisms by which EVs mediate communication between cells and their potential applications, scientists can develop new strategies to improve patient care and outcomes.
In conclusion, the study of extracellular vesicles holds great promise for revolutionizing medical advancements and improving patient care. As research continues to unlock the potential of these tiny structures, we can look forward to new insights into cellular communication, disease progression, and innovative therapeutic strategies.
Frequently Asked Questions
What are the main types of extracellular vesicles?
How do extracellular vesicles facilitate intercellular communication?
What is the role of tumor-derived EVs in cancer progression?
How can EVs be used as biomarkers for early disease detection?
What are the potential therapeutic applications of EVs?
About the Author
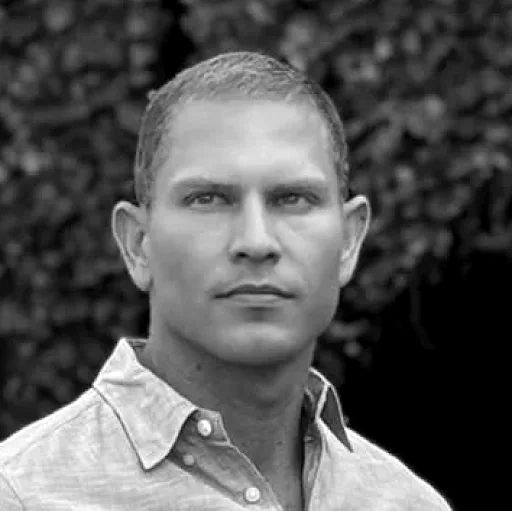
Bradley Keys
At Targeted Bioscience, Bradley delves into the forefront of precision medicine and biotechnology. His writing reflects the company's commitment to advancing personalized healthcare through innovative solutions, particularly in oncology. Bradley's work aligns with Targeted Bioscience's mission to develop cutting-edge tools and therapies that enhance disease treatment accuracy and efficacy.
Comments